© 2022 MJH Life Sciences and Chromatography Online. All rights reserved.
© 2022 MJH Life Sciences™ and Chromatography Online. All rights reserved. Fumed Silica Buy
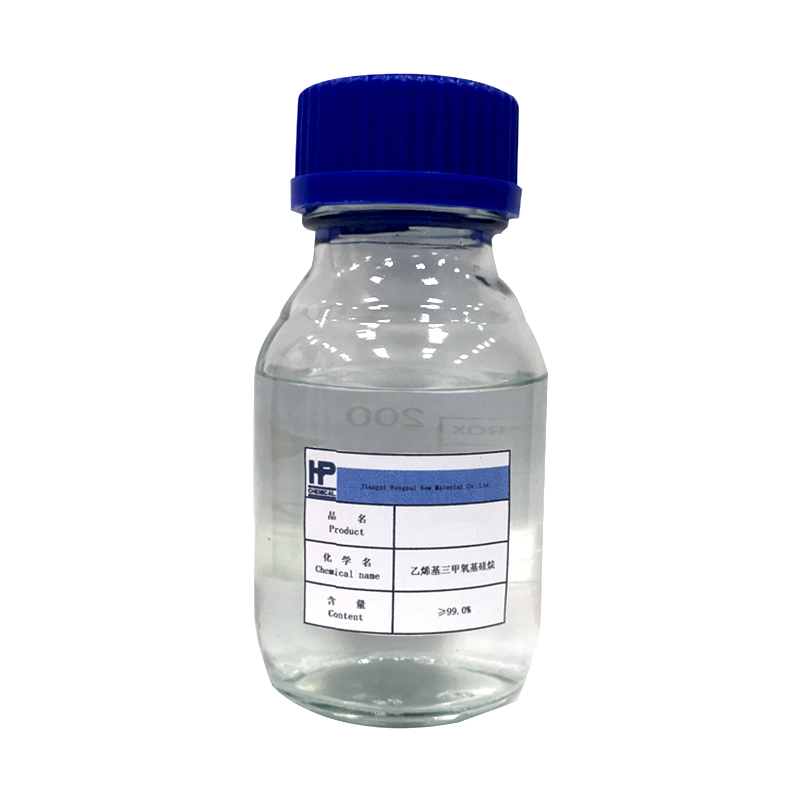
Porous layer open tubular (PLOT) columns are traditionally built with particles that are adhered to the tubing walls. These columns have unique selectivity and provide a great alternative when gaseous samples need to be separated, but these columns also have been used to separate higher boiling point analytes. There are many different commercially available stationary phases of PLOT columns, including alumina-based columns, molecular sieves, and porous polymers. Alumina-based columns have an aluminum oxide stationary phase that is then deactivated with different salts. These columns have high capacity, superior loading ability, and produce symmetrical peaks. Molecular sieve columns are designed specifically for permanent gas separations because the columns have high retention. Porous polymer columns are highly hydrophobic, making them more applicable to analyzing a wider range of samples.
There are a wide variety of commercially available stationary phases of porous layer open tubular (PLOT) columns (Table I), such as alumina, molecular sieve, carbon, silica, and a variety of porous polymers. In general, PLOT columns are traditionally built with particles that are adhered to the tubing walls.
Alumina columns have an aluminum oxide stationary phase that is then deactivated with various salts. These columns have high capacity and produce symmetrical peaks. Molecular sieve columns are designed specifically for permanent gas separations. Carbon-layer columns provide selectivity for inorganic and organic gases, like separating carbon monoxide and carbon dioxide. Silica columns are a great alternative to packed columns because they are made to be selective for C1–C4 compounds. Porous polymer columns are hydrophobic, which makes them more applicable to analyzing a wider range of samples. DVB/vinylpyridine polymers are moderately polar and DVB/ethylene glycol/dimethacrylate are the most polar polymer columns. These commercially available PLOT columns (Table I) are extensively tested to produce superior separations and are made to not shed particles, which was previously a concern with PLOT columns (1). Although PLOT columns have been primarily applied in gas chromatography (GC) with the main focus on separating low boiling point analytes (2,3), there is evidence of PLOT column use beyond light samples (4–6). The versatility of PLOT columns has been exploited even further by their use in dynamic vapor microextraction (DVME) (7–9), and even in liquid chromatography (LC) (10–12). Here, we discuss the application of PLOT columns beyond their original primary use for permanent gaseous sample separations.
The first laboratory-made PLOT columns were introduced in 1963 (13,14). Since that time, these columns have been mostly used for separating light mixtures like permanent gases (2,15,16) or light hydrocarbons (3,17,18). It has been shown that PLOT columns are very retentive for light compounds. For example, a 10-m molecular sieve column has been used to separate permanent gases in the presence of hydrocarbons and nitriles (2). With the exception of helium and neon (which were coeluted), all the other permanent gases (argon, dinitrogen, krypton, xenon), along with methane and carbon monoxide, were baseline separated (2). Alumina columns have been evaluated by Ji and others, where they showed that these columns are highly retentive for C1–C6 compounds (3). The baseline separation of methane, ethane, ethylene, propane, and higher carbon content compounds was achieved using a 30-m alumina column (3). Retentiveness of PLOT columns that was obtained with light compounds could potentially be applied to higher boiling point analytes and there has been some work done throughout the years to broaden the use of PLOT columns.
In 1974, fast, high temperature separations were performed using a molecular sieve column by Soulages and associates where the paraffin and naphthalene content was investigated in saturated hydrocarbon distillates (19). Arpino and colleagues in 1977 presented a new PLOT column that was made with a graphitized thermal carbon-black polar stationary phase that could be used for GC and LC (20). This column was used to investigate two oxidation reactions, where citral (boiling point: 225 °C) was produced from geraniol (boiling point: 230 °C) (20). Reaction products were separated, and more than 30 by-products were identified (20). Another application, reported in 1980 by Uden and others, involved separating volatile metal chelates and organometallic compounds (like chromium trifluoroacetylacetonate, chromium hexatluoroacetylacetonate-trifluoroacetylacetonate che- lates, copper (II) tetradentate p-ketoamine chelates, and cyclopentadienylmanganese tricarbonyl compounds) using carborane-silicone Dextil PLOT stationary phases in a stainless-steel column (21). Following that, in 1983, Nijs and associates implemented alumina-coated PLOT columns to separate hydrocarbons up to C10 (4). In the same study, the authors demonstrated a beautiful separation of naphtha, with p-xylene (boiling point: 138 °C) being the heaviest compound present (4). Another report, where some higher boiling point analytes were separated in conjunction with permanent gases, was published by Greally and colleagues in 1998 (5). In this study, a novel PLOT column with a hydrophobic silica layer coated on a fused-silica capillary was used (5). Various halogenated hydrocarbons were separated in this study; although most analytes had a boiling point <0 °C, they also separated heavier halogenated hydrocarbons, like chloroform (boiling point: 61 °C) (5).
Another more recent work involving hydrocarbon mixture separations was reported by Wawrzyniak and others while evaluating a novel stationary phase of 4,4,4-trifluoro-1-phenyl-1,3-ketoiminepropyl silane used as a porous layer bonded on to 5-μm nucleosil silica (22). Halogenated hydrocarbons up to C6 were analyzed, which facilitated the separation of the heaviest compound in this study of 1,2-dichlorobenzene (BP: 180 °C) (22). Another unique application of PLOT columns includes isotopic separations where a commercially available porous polymer PLOT column has been used to separate acetaldehyde and methanol from their deuterated isotopologues, performed using headspace sampling (6).
Implementation of PLOT columns has also emerged in the field of comprehensive two-dimensional gas chromatography (GC×GC), where the complementary separation mechanisms provided by two different PLOT columns can be leveraged to produce intriguing 2D separations. Light hydrocarbons and some solvents were separated using different commercially available PLOT columns by Patrushev and associates in 2018, with the heaviest compound separated being methylcyclohexane (boiling point: 101 °C) (23). GC×GC has also been shown to provide informative 2D separations for gasoline, which we described in our recent report, where a distinct commercially available PLOT column was applied for each dimension. On the first dimension (1D), a silica PLOT column was applied, while on the second dimension (2D), a porous polymer PLOT column was used (24).
PLOT columns have unique selectivity and provide a great alternative to wall-coated open tubular (WCOT) columns or packed columns for separations of light samples (25–27). Environmental Protection Agency (EPA) Appendix IX volatiles calibration megamix was separated by GC using the same temperature program and chromatographic conditions on a WCOT column (Rtx-200) in Figure 1a, and on a PLOT-Q column in Figure 1b. Most of the mix eluted at the beginning of the chromatogram using a WCOT column; while using the PLOT-Q column, the entire mixture was well retained and separated, everything from carbon disulfide (boiling point: 46 °C) to naphthalene (boiling point: 218 °C). In this example, even a very short length PLOT column provides unique selectivity for low boiling point analytes relative to the selectivity that is provided using a WCOT column. A heavier sample of gasoline was used to further show the unique separation abilities of PLOT columns. Gasoline is one of the heaviest “common” samples that can be run on PLOT columns. Figure 2 presents optimized gasoline separations on a porous polymer (PLOT-S) column (Figure 2a) and an alumina oxide (Rt-Alumina BOND/MAPD) column (Figure 2b). The heaviest compound that elutes from both columns is 1-methyl naphthalene, which has a boiling point of 244 °C. Although both columns provide intriguing separations of gasoline with relatively short run times, Rt-Alumina BOND/MAPD provides a better separation of very similar molecules. As shown in Figures 2c and 2d, neopentane, 1-pentene, isopentane, and pentane are separated into four peaks on Rt-Alumina BOND/ MAPD (Figure 2d), whereas on PLOT-S column (Figure 2c), these compounds overlap into two peaks. Rt-Alumina BOND/ MAPD also provides a mix of sharper and broader peaks. These separations show the ability to apply PLOT columns for samples containing compounds with a wide variety of boiling points—for example, isobutane (boiling point: -11 °C) to 1-methyl naphthalene (boiling point: 244 °C).
More recently, PLOT columns have also been used for cryoadsorption, which was developed at the National Institute of Standards and Technology (NIST) in 2009 by Bruno and colleagues (7). This technique is also referred to as dynamic vapor microextraction (DVME). More specifically, it is an ultrasensitive, low volume purge-and-trap sampling method where vapor-phase analytes are concentrated on a section of the PLOT column (9). DVME is a dynamic headspace sampling technique that is especially useful when low volatility compounds are of interest.
A cryoadsorption apparatus for DVME adapted from the pioneering report by Bruno and colleagues is shown in Figure 3a (7). In this work, the apparatus was equipped with a short alumina PLOT column as a purge trap (Figure 3b) operating at a low temperature. The apparatus was evaluated using a pure explosive compound 2,3,4-trinitrotoluene as well as a practical explosive C-4 (7). Later, the same group published a broader study using alumina-coated PLOT columns for cryoadsorption where industrial and military plastic bonded explosives (PBX) tagged C-4, Semtex-1A, Semtex-H, detonating cord (detcord), and sheet explosive (Detaflex), were investigated (28). DVME was adapted later by Nichols and others. In this study, short PLOT columns were used in cryoadsorption on arson fire debris samples (29).
Most recently, cryoadsorption was adapted to develop a portable vapor sampling device. The first account of such work was published in 2016 by Bruno and colleagues, where the instrument was laboratory tested using naphthalene, kerosene, and other hydrocarbons, producing accurate detection and quantitation after a short collection time of 3 s with a concentration limit of detection (LOD) of less than 1 ppm (8,30). Then, this device was in-field tested by Harries and associates in 2019 on an old U.S. Army communication bunker as a simulated shipping container (31). This device was able to detect naphthalene, two-explosive-related compounds, four protein decomposition markers, and gasoline (31). This work was further expanded on by another study that examined how long after in-field collection the samples stay preserved when collected on the PLOT column. It was determined that the integrity of the samples was fully preserved for 24 h, with losses occurring by the seventh day (9).
DVME has been shown to be a very sensitive technique. Burger and others examined the difference between natural gas sampling using multi-PLOT-cryo (Figure 3c) and a direct injection of the same amount of natural gas using a gas-tight syringe (Figure 3d). The study showed how much more sensitive and suitable for the analysis of trace components the PLOT-cryo technique is over traditional sampling methods (32). Because of the superior sensitivity of DVME, it has been used in recent years to analyze a broad range of samples: fuels (33), cannabinoids (34), and forensics (28,29,35,36).
Traditionally, LC is performed using packed columns with bonded phases, but it has been shown that open tubular columns can provide higher column efficiency (37). There have been a few studies into using open tubular columns in LC in the 1970s and 1980s (38,39), and it has been shown that to reach column efficiencies close to what is achieved in GC, the columns must be not more than 15 μm in inner diameter (40). Open tubular column use was revitalized in LC after coupling to electrospray (ES) mass spectrometry (MS) (41).
PLOT columns have become increasingly popular in LC in recent years. Most papers have focused on using PLOT columns for LC separations of various biological samples. In 2010, Rogeberg and associates separated intact proteins in skim milk using polystyrene divinylbenzene PLOT columns (10); narrow peaks (0.144–0.33 min) and good within-column repeatability in retention times (0.6% RSD) were achieved, with less than 1.1% carryover injection-to-injection. In 2011, Wang and colleagues analyzed haptoglobin from lung cancer patients with a 10 μm i.d. polystyrene-divinylbenzene PLOT column by LC coupled to collision-induced dissociation/electron transfer dissociation–MS (LC−CID/ETD-MS) (11). Separation of three glycopeptides (T1, T2, and T3) was performed, whereby the intensity of a 10-fmol sample separation using the PLOT column was similar to that of a 200-fmol sample separation when a standard packed column was used for LC–MS (11). A new zwitterionic PLOT column was introduced by Peng and others in 2016 that was used to separate flavonoids from rootstock of licorice (42). Good RSD for differences in retention time run-to-run (≤1.1%), day-to-day (≤0.78%), and column-to-column (≤1.1%), were obtained.
There have also been nano-LC and pico-LC systems that have been introduced in the recent years, where a PLOT column was used for the separations. In 2009, a nano-LC system using an amine-bonded poly(vinylbenzyl chloride-divinylbenzene) hydrophilic interaction LC (HILIC) PLOT column was used for the separation of a glycan mixture, and only 50 fmol of a dextran ladder was needed to identify dextrans up to G22 (43). In 2011, Thakur and associates presented a workflow where breast cancer cells were analyzed using PLOT-LC tandem MS (MS/MS), demonstrating good quantitative reproducibility between the same patient samples (44). In 2017, Li and colleagues presented a new pico-PLOT HPLC system that was used to separate amino acid enantiomers (45). A very recent report from Vargas Medina and others in 2022 describes the development of nano-LC coupled to electron ionization (EI)–MS. Polyaromatic hydrocarbons and thermolabile pesticides were separated, and the LOD of such a system was determined to be 25 μg/L (12).
PLOT columns provide unique retention and selectivity for low boiling point analytes relative to WCOT columns (25–27). Although most of their use has been shown with light mixtures (2,3), there has been some work done to broaden their use throughout the years (4–6). We demonstrated their unique abilities to separate light compounds as well as heavier boiling point analytes (Figures 1 and 2), which shows a broad analyte range that can be analyzed using PLOT columns. As in one-dimensional (1D) chromatography, GC×GC using PLOT columns has mostly been employed to separate samples containing light boiling point compounds and continues to be an underutilized application area for PLOT columns. Further applications of PLOT columns have been shown by their use in a new sampling technique of dynamic vapor microextraction (DVME), and this technique has been applied to analyze various samples, including explosives, fuels, and cannabinoids (33–35). PLOT columns have also gained attention in the LC community because of their higher efficiency, where they have been adapted to be used in nano-LC systems to analyze mostly biological samples (10–12).
It is important to note that because there are no commercially available PLOT-LC columns, this is a research area under development with ample opportunities. Broader use of PLOT columns is a growing research area, especially with their use for heavier samples in GC. Applying these columns for sampling using dynamic vapor microextraction and utilizing their better column efficiency in liquid chromatography also demonstrate expanding utility of the technology.
(1) “Restek’s PLOT Column Family,” Restek Corporation (Bellefonte, PA, 2020).
(2) E. de Vanssay, P. Capilla, D. Cos- cia, L. Do, R. Sternberg, and F. Raulin, J. Chromatogr. A 639, 255–259 (1993). https://doi.org/10.1016/00219673(93)80261-6
(3) Z. Ji and I.L. Chang, J. High Resolut. Chromatogr. 19, 32–36 (1996). https://doi.org/10.1002/jhrc.1240190106
(4) R.C.M. De Nijs and J. De Zeeuw, J. Chromatogr. A 279, 41–48 (1983). https://doi.org/10.1016/S0021-9673(01)93598-8
(5) B.R. Greally, G. Nickless, P.G. Sim- monds, M. Woodward, and J. de Zeeuw, J. Chromatogr. A 810, 119–130 (1998). https://doi.org/10.1016/S00219673(98)00240-4
(6) H.-G. Schmarr, M. Wacker, and M. Mathes, J. Chromatogr. A 1481, 111– 115 (2017). https://doi.org/10.1016/j.chroma.2016.12.023
(7) T.J. Bruno, J. Chromatogr. Sci. 47, 569–574 (2009). https://doi.org/10.1093/chromsci/47.7.569
(8) T.J. Bruno, J. Chromatogr. A 1429, 65–71 (2016). https://doi.org/10.1016/j.chroma.2015.12.013
(9) M.E. Harries and K.M. Jeerage, J. Chromatogr A 1660, 462670 (2021). https://doi.org/10.1016/j.chroma.2021.462670
(10) M. Rogeberg, S.R. Wilson, T. Greibrokk and E. Lundanes, J. Chromatogr. A 1217, 2782–2786 (2010). https://doi.org/10.1016/j.chroma.2010.02.025
(11) D. Wang, M. Hincapie, T. Rejtar, and B.L. Karger, Anal. Chem. 83, 2029– 2037 (2011). https://doi.org/10.1021/ac102825g
(12) D.A. Vargas Medina, J.S. da S. Burato, J.V.B. Borsatto, and F.M. Lanças, J. Chromatogr. A 1674, 463143 (2022). https://doi.org/10.1016/j.chroma.2022.463143
(13) J.J. Kirkland, Anal. Chem. 35, 1295–1297 (1963). https://pubs.acs.org/doi/pdf/10.1021/ac60202a038
(14) D.L. Petitjean and C.J. Leftault, Jr., J. Chromatogr. Sci. 1, 18–21 (1963). https://doi.org/10.1093/chrom-sci/1.3.18
(15) H. Yun and M.L. Lee, Field Anal. Chem. Technol. 1, 60–64 (1996). https://doi.org/10.1002/(SICI)1520-6521(1996)1:1<60::AID-FACT8>3.0.CO;2-I
(16) H. Yun, M.L. Lee, and K.E. Markides, J. Microcolumn Sep. 7, 207–212 (1995). https://doi.org/10.1002/mcs.1220070304
(17) W.-C. Liao, C.-F. Ou-Yang, C.-H. Wang, C.-C. Chang and J.-L. Wang, J. Chromatogr. A 1294, 122–129 (2013). https://doi.org/10.1016/j.chroma.2013.04.008
(18) G.L. Reid III and D.W. Armstrong, J. Microcolumn Sep. 6, 151–157 (1994). https://doi.org/10.1002/mcs.1220060208
(19) N.L. Soulages and A.M. Brieva, J. Chromatogr. A 101, 365–371 (1974). https://doi.org/10.1016/S0021-9673(00)82853-8
(20) P. Arpino, C. Vidal-Madjar, G. Guiochon and S. Békássy, J. Chromatogr. A 138, 173–182 (1977). https://doi.org/10.1016/S0021-9673(00)98008-7
(21) P.C. Uden, D.E. Henderson, F.P. DiSanzo, R.J. Lloyd, and T. Tetu, J. Chromatogr. A 196, 403–414 (1980). https://doi.org/10.1016/S0021-9673(00)84743-3
(22) R. Wawrzyniak and K. Adamska, J. Chromatogr. A 1249, 215–225 (2012). https://doi.org/10.1016/j.chroma.2012.06.025
(23) Y.V. Patrushev and V.N. Sidelnikov, J. Chromatogr. A 1579, 83–88 (2018). https://doi.org/10.1016/j.chroma.2018.10.015
(24) L. Mikaliunaite, P.E. Sudol, C.N. Cain, and R.E. Synovec, J. Chromatogr. A 1652, 462358 (2021). https://doi.org/10.1016/j.chroma.2021.462358
(25) C.-H. Wang, C.-Y. Huang, H.-K. Yak, H.-C. Hsieh, and J.-L. Wang, Chemosphere 264, 128504 (2021). https://doi.org/10.1016/j.chemosphere.2020.128504
(26) P. Kotnik, M. Čolnik, M. Finšgar, Ž. Knez, and M. Škerget, Polym. Degrad. Stab. 182, 109386 (2020). https://doi.org/10.1016/j.polymdegradstab.2020.109386
(27) E.Y. Yakovleva and Y.V. Patrushev, Chromatographia 84, 1095–1104 (2021). https://doi.org/10.1007/s10337-021-04092-1
(28)T.M. Lovestead and T.J. Bruno, Anal. Chem. 82, 5621–5627 (2010). https://doi.org/10.1021/ac1005926
(29) J.E. Nichols, M.E. Harries, T.M. Lovestead, and T.J. Bruno, J. Chromatogr. A 1334, 126–138 (2014). https://doi.org/10.1016/j.chroma.2014.01.080
(30) M. Harries, S. Bukovsky-Reyes, and T.J. Bruno, J. Chromatogr. A 1429, 72–78 (2016). https://doi.org/10.1016/j.chroma.2015.12.014
(31) M.E. Harries and T.J. Bruno, Forensic Chem. 16, 100182 (2019). https://doi.org/10.1016/j.forc.2019.100182
(32) J.L. Burger, T.M. Lovestead, and T.J. Bruno, Energy Fuels 30, 2119–2126 (2016). https://doi.org/10.1021/acs.energyfuels.6b00043
(33) M.E. Harries, S.S. Wasserman, J.L. Berry, and K.M. Jeerage, Forensic Chem. 22, 100301 (2021). https://doi.org/10.1016/j.forc.2020.100301
(34) T.M. Lovestead and T.J. Bruno, Forensic Chem. 5, 79–85 (2017). https://doi.org/10.1016/j.forc.2017.06.003
(35) T.M. Lovestead and T.J. Bruno, Food Chem. 121, 1274–1282 (2010). https://doi.org/10.1016/j.foodchem.2010.01.044
(36) T.M. Lovestead and T.J. Bruno, Forensic Sci. Int. 204, 156–161 (2011). https://doi.org/10.1016/j.forsciint.2010.05.024
(37) K. Göhlin, A. Buskhe, and M. Larsson, Chromatographia 39, 729–739 (1994). https://doi.org/10.1007/BF02274590
(38) T. Tsuda and M. Novotny, Anal. Chem. 50, 632–634 (1978). https://doi.org/10.1021/ac50026a023
(39)T. Tsuda, K. Nomura, and G. Nakagawa, J. Chromatogr. A 248, 241–247 (1982). https://doi.org/10.1016/S0021-9673(00)87275-1
(40)G. Guiochon, in High-Performance Liquid Chromatography, C. Horváth, Ed. (Academic Press, Cambridge, Massachusetts, 1980), pp. 1–56.
(41) G. Hulthe, M.A. Petersson, and E. Fogelqvist, Anal. Chem. 71, 2915– 2921 (1999). https://doi.org/10.1021/ac981352f
(42) L. Peng, M. Zhu, L. Zhang, H. Liu, and W. Zhang, J. Sep. Sci. 39, 3736– 3744 (2016). https://doi.org/10.1002/jssc.201600535
(43) Q. Luo, T. Rejtar, S.-L. Wu, and B.L. Karger, J. Chromatogr. A 1216, 1223– 1231 (2009). https://doi.org/10.1016/j.chroma.2008.09.105
(44)D. Thakur, T. Rejtar, D. Wang, J. Bones, S. Cha, B. Clodfelder-Miller, E. Richardson, et al, J. Chromatogr. A 1218, 8168–8174 (2011). https://doi.org/10.1016/j.chroma.2011.09.022
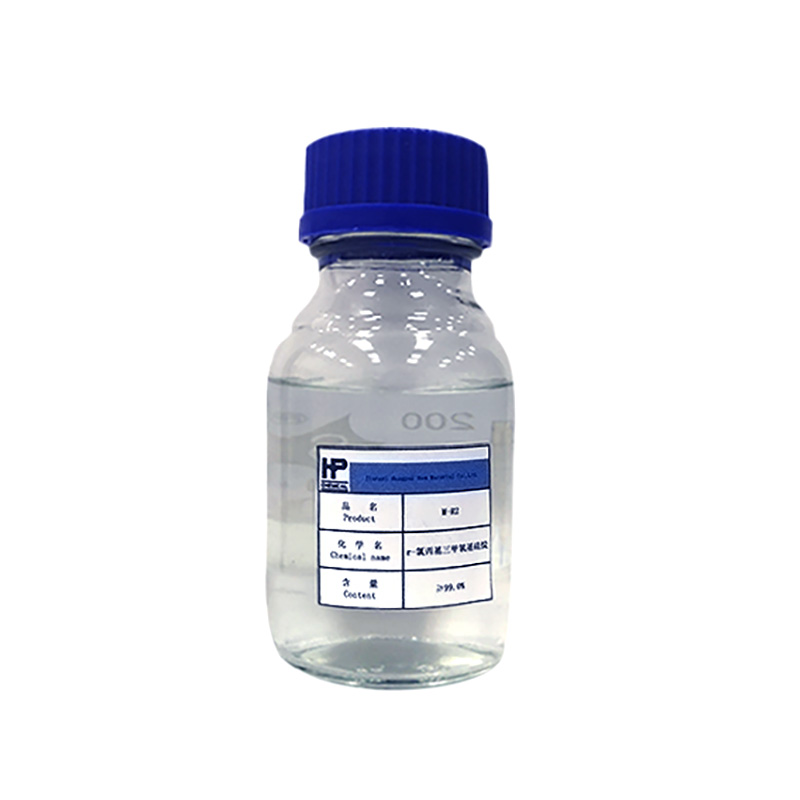
Glutathione Booster (45) R. Li, Y. Shao, Y. Yu, X. Wang, and G. Guo, Chem. Commun. 53, 4104– 4107 (2017). https://doi.org/10.1039/C7CC00799J